Graphene
the differentiating material for the use of solar energy
Solar energy, being a clean and abundant source, is one of the best renewable energy options. However, despite technological advances, its utilization remains insignificant to date. According to statistics, only 0.015% of solar energy is used for electricity production, 0.3% for heating, and 11% for natural biomass photosynthesis. In contrast, about 85% of global energy needs are met through fossil fuels, which we know are finite and highly polluting resources.
“In 90 minutes, the sun sends enough energy to Earth to satisfy the entire planet’s energy demand for a year”
Solar cells are devices that convert solar energy into electricity through the photovoltaic effect. They are made of semiconductor materials that produce an electric field when exposed to sunlight and are divided into four generations:
First Generation
First-generation solar cells were first manufactured in 1954 by Bell Laboratories. They used crystalline films of monocrystalline and polycrystalline silicon with an average thickness of 200 to 300 µm, initially achieving an energy conversion efficiency of 6%, which later rose to 29% with the use of gallium arsenide (GaAs). In fact, these types of cells remain the most popular thanks to their high absorption coefficient.
Second Generation
It employs first-generation cells in conjunction with a new series of considerably thinner films of only 10 µm thickness based on microcrystalline silicon (µC-Si), amorphous silicon (A-Si), copper indium gallium selenide (CIGS), and cadmium telluride/cadmium sulfide (CDTE/CDS). Two advantages of this generation are its cost and mechanical resistance, while its disadvantage is that to achieve these benefits, conversion efficiency had to be sacrificed to some extent, reducing to 23%. Despite this drawback, these types of solar cells are still available in the market.
Third Generation
The high manufacturing cost of silicon cells, resulting from their complex manufacture from high-quality silicon, paved the way for the third generation, which integrates more flexible, lightweight, and economical materials. This is how dye-sensitized solar cells (DSSC), perovskite solar cells (PSC), organic/polymeric solar cells (OPV), quantum dot-sensitized solar cells (QDSSC), and finally, multi-junction solar cells emerged.
Probably the most interesting but also the most complex and expensive cell in this category is the multi-junction cell. As its name suggests, it consists of multiple junctions from various semiconductor materials that produce an electric current in response to different incident wavelengths, thus improving the conversion of sunlight into electricity; so far, the conversion efficiency recorded with these designs is 36%.
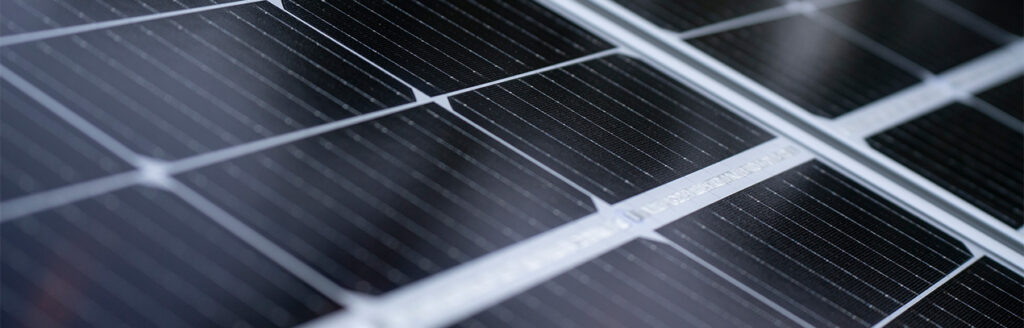
Fourth Generation (Hybrid)
This latest generation fuses the flexibility and low cost of polymers with the stability and durability of nanoparticles and metal oxides, as well as carbon nanostructures like graphene.
Graphene Solar Cells
Graphene is a carbon nanostructure with high conductivity, transmittance, mechanical strength, thermal stability, and chemical inertness. It also has a zero band gap structure that allows electron conduction as if it were a metal, as well as the quantum Hall effect that allows its free charges to move easily in two dimensions at high speed.
Thanks to these characteristics, it was discovered that graphene can be used for the manufacture of transparent conductive electrodes, energy harvesting devices, photodetectors, and other optical devices. However, although graphene is an excellent conductor, it does not have the same capacity to collect the electric current produced within a solar cell, unlike its oxidized variant, graphene oxide (GO), which is a less conductive material but more transparent and a better charge collector.
“Graphene has been classified as a semimetallic semiconductor that presents linear electronic dispersion with high mobility and high speeds.”
Another important factor of graphene is its thickness, which in turn depends on its number of layers. For this reason, graphene is classified as monolayer, bilayer, trilayer, few-layer (<5 layers), and multilayer (<10 layers), remembering that more than 10 layers of graphene is already considered graphite. As bilayer and trilayer graphene maintain a better balance between their transmittance and resistance properties, they are the most suitable for use in solar cells, on which ideally a maximum thickness of 20 nm should be maintained.
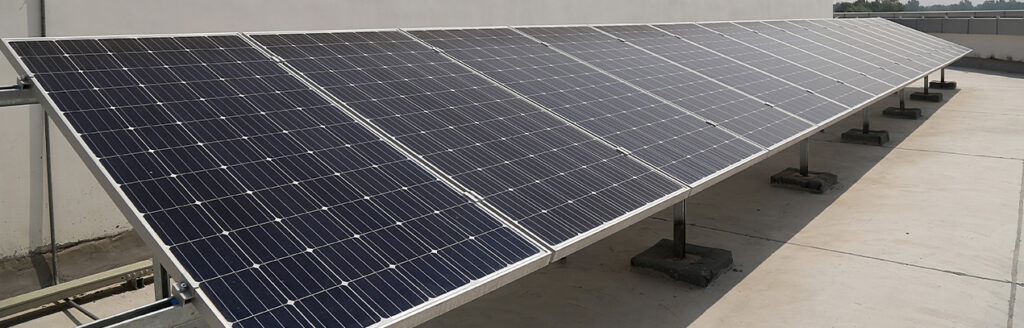
Applications of Graphene on Solar Cell Components
Transparent Conductive Electrodes (TCE)
The first components in which graphene has shown beneficial impacts are transparent conductive electrodes (TCE). Previously, due to its high conductivity and transmittance in the visible spectrum, indium tin oxide (ITO) was used for TCEs. However, the films tend to be fragile and unstable at high temperatures. Additionally, it’s worth mentioning that indium is an extremely scarce, toxic, and expensive metal. In fact, as the demand for solar cells increased, the price of indium rose to such an extent that the cost of TCEs represented 50% of manufacturing costs. For these reasons, ITO was replaced by fluorine-doped tin oxide (FTO), which is more economical and withstands aggressive chemical treatments at high temperatures.
In addition to FTO, graphene appears as an alternative to overcome the limitations of ITO in solar cell TCEs, as long as the ratio between resistance and transmittance is increased. For this, chemical doping and co-doping of graphene with polymers such as PEN, PEDOT:PSS, gold nanoparticles, silver nanowires, cubic platinum nanoparticles, nitric acid (HNO₃), thionyl chloride (SOCl₂), triethylenetetramine (TETA), graphene oxide (GO), and bis(trifluoromethanesulfonyl)imide (TFSA) have been studied with good results.
Doping: chemical modification to decrease graphene resistance and expand work function.
HNO₃-AuNp: nitric acid-gold nanoparticles.
PEN: poly(ethylene naphthalate): polyester polymer with barrier properties.
PEDOT:PSS: Poly(3,4-ethylenedioxythiophene)-poly(styrene sulfonate) / transparent and conductive polymer.
Photoactive Layers
The photoactive layers of solar cells include active interfacial layers, electron/hole charge separation layers, electron/hole transport layers (ETL/HTL), electron/hole blocking layers, and buffer layers.
According to reports, lithium-neutralized graphene oxide (GO-Li) in interfacial layers improves not only efficiency but also device stability in weathering (heat, air, and humidity) or as an anti-reflective protective film, thanks to its chemical inertness and transparency. Other functionalizations of graphene for photoactive layers include thiolate-reduced graphene oxide (TrGO) and graphene with cadmium sulfide (CdS).
Areas of Opportunity for Graphene on Different Types of Solar Cells
Silicon Solar Cells
Research indicates that the conversion efficiency of graphene solar cells can be improved by incorporating a dielectric passivation layer between the graphene and the silicon substrate to suppress electron diffusion from the latter to the graphene layer. Among the insulating materials that could be used effectively are silicon dioxide (SiO₂), molybdenum disulfide (MoS₂), aluminum oxide (Al₂O₃), graphene oxide (GO), hexagonal boron nitride (h-BN), poly(3-hexylthiophene-2,5-diyl) (P3HT), quantum dots, molybdenum trioxide (MoO₃), and spiroOMeTAD, to name a few.
Organic/Polymeric Solar Cells
In this type of cell, graphene can have three general functions:
1. As an additive in donor or donor-acceptor materials,
2. As a transparent conductive electrode (anode and cathode),
3. As a separate photoactive layer.
Bilayer and trilayer graphene, thanks to their high conductivity, can correct the charge transport problems of the electron donor-acceptor system (P3HT:PCBM) associated with the imbalance of electron and hole mobility to avoid charge trapping and improve efficient collection.
P3HT:PCBM electron donor-acceptor system of polymeric solar cells.
P3HT: conductive polymer
PCBM: fullerene derivative.
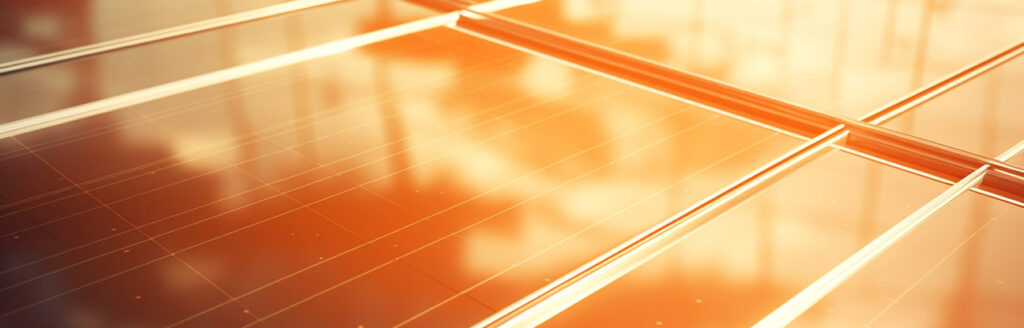
Dye-Sensitized Solar Cells
This technology tries to emulate the process of plant cells to produce energy from organic pigments. In them, graphene was initially used to replace FTO in photoanodes, but over time additional advantages were identified in the following components:
- Photoanodes: as a transparent conductor for both titanium dioxide (TiO₂) and pigment sensitization; in photoanodes, graphene can improve the charge transport rate, prevent recombination, and increase light capture.
- Counter electrodes: as a substitute for platinum,
- As a photoanode additive: to improve electron transfer,
- Polymer electrodes, in which a polymer (PEDOT-PSS) allows conductivity, while graphene facilitates catalysis.
Photoanode: It is the vehicle for electrons from the photoexcited pigment to the external circuit. It consists of a layer of titanium dioxide (TiO₂) on a conductive glass or plastic substrate.
Counter electrodes: participate in the injection of electrons from the photooxidized pigment into the electrolytes to catalyze reduction reactions.
Perovskite Solar Cells
Perovskite is a mineral composed of calcium and titanium oxide that has been used for the manufacture of solar cells since 2009. Initially, its efficiency was 3.9% but quickly rose to 32%. Despite its good performance, ease of manufacture, and versatility, perovskite solar cells have two major disadvantages. The first is easy degradation in weathering, and the second is toxicity related to the presence of lead, which naturally raises concerns for human and environmental health. It is then that efforts to counteract these drawbacks have focused, on the one hand, on chemically modifying the mineral and, on the other, on encapsulating it to protect it from external conditions.
As with other types of cells, it has also been identified that the presence of graphene or its reduced variant within the photoactive layers (HTL/ETL) of perovskite cells can further improve their efficiency by 14 to 28%. This is because graphene, being an ambipolar material, that is, it can move charges in different directions, can help balance the work function – conductivity, speed up electron extraction, and improve stability in weathering.
Clearly, and as mentioned previously, the functionalization or doping of graphene plays an important role in improving the performance of solar cell components. In the case of perovskite cells, functionalization with metal nanoparticles, metal oxides, and/or perovskite nanoparticles is useful, not only to improve the stability of graphene but to increase the surface area and electrical conductivity throughout the system.
While it is a fact that graphene solar cells are not yet commercially available, some advances have already been reported in this direction. The first of these is the G12 Evolution series from Znshine Solar, composed of three graphene modules which, in 2018, won a contest to provide 37.5 MW of modules to Bharat Heavy Electricals Limited (BHEL), India’s largest power generation equipment manufacturer. According to the contract, 10% of the shipment was graphene-coated solar panels. Subsequently, in 2019, the company signed a contract with Etihad Energy Services of the United Arab Emirates for the supply of 100MW.
Finally, at the end of 2024, the Australian companies Halocell Energy and First Graphene announced an alliance for a two-year project for the manufacture of perovskite solar cells with graphene. The objective was to accelerate the manufacturing process, improve light capture performance, and thus expand production and meet commercial demand. According to published information, graphene perovskite modules are up to five times more efficient and cost-effective than common silicon cells.
Bibliography
- Photovoltaic Cell Generations and Current Research Directions for Their Development Materials 2022, 15, 5542;
- Recent Advancements in Applications of Graphene to Attain Next-Level Solar Cells. C 2023, 9, 70;
- Rational and key strategies toward enhancing the performance of graphene/silicon solar cells. Mater. Adv., 2023, 4, 1876;
- Recent Applications of Graphene in Dye-sensitized Solar Cells. Current Opinion in Colloid & Interface Science 20 (2015) 406;
- Recent advances of graphene-based materials in planar perovskite solar cells. Next Nanotechnology 5 (2024) 100061;
- https://www.halocell.energy/news-posts/first-graphene-to-supply-halocells-indoor-perovskite-solar-cell-production-line;
- https://www.graphene-info.com/graphene-solar-panels