Carbonation and Graphene Oxide:
A Solution for Reducing CO₂ Emissions
In previous articles, we discussed the cement industry’s impact on CO₂ emissions and the commitments made to reduce them by 2050. Today, we explore how carbonation—a process generally seen as a concrete pathology—could help offset some CO₂ emissions from cement production.
What is Carbonation?
In concrete, carbonation is a natural process where CO₂ from the environment reacts with moisture in the concrete, converting the alkaline calcium hydroxide in cement paste to calcium carbonate with a more neutral pH. This reaction lowers the concrete’s pH from around 12–13 to approximately 9, exposing steel reinforcements to corrosion.
What Affects Carbonation?
Carbonation rate depends on the diffusion of CO₂ and its reactivity with the cement matrix, which is in turn influenced by the matrix’s microstructure, hydration products (calcium hydroxide, calcium silicate hydrate, alkaline oxides, etc.), and pore structure (distribution, size, and saturation). Therefore, carbonation proceeds more slowly in low-permeability or dry concretes than in permeable ones with 50–60% humidity. To reduce porosity and calcium hydroxide levels, micrometric additives like fly ash, blast furnace slag, metakaolin, silica fume, and some nanomaterials are used during concrete production, alongside practices like applying surface coatings.
Carbonation as an Emission Reduction Tool
Carbonation can be viewed in two ways: first, as a concrete pathology, and second, as a CO₂-reducing opportunity. There are two types of carbonation: natural and accelerated. Natural carbonation is slow and does not capture CO₂, while accelerated (or mineral) carbonation uses high CO₂ concentrations, speeding up cement hydration and producing carbonates in which CO₂ is permanently stored in a thermodynamically stable mineral form. This process, known as recarbonation, involves the same carbonate used as a raw material in cement production. Companies like Blue Planet, Carbon Cure, Solidia Technologies, and Carbi Crete are developing strategies to sequester up to 17 kg of CO₂ per cubic meter of prefabricated concrete, as this process requires controlled conditions.
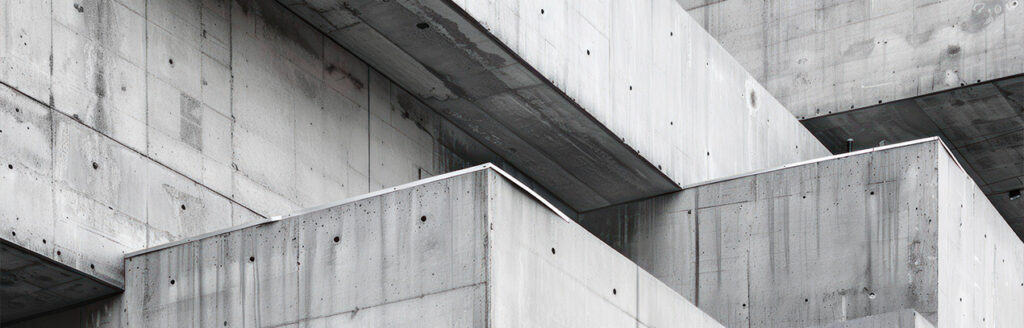
Graphene Oxide (GO) and Its Impact
Graphene oxide (GO) is a carbon nanostructure whose multifunctionality offers numerous benefits across industries. In concrete, GO enhances mechanical strength and durability, though its effects on carbonation and CO₂ capture are less well-documented.
Research conducted by the University of Arlington, Texas, in 2022 examined GO’s interaction mechanism in concrete cured under accelerated carbonation. Results indicated that GO, by improving cement hydration, refines concrete pores with calcium carbonate precipitated on hydration products and cement particles, limiting chemical reactions between hydration products and CO₂ under continuous CO₂ flow. The study concluded that GO not only enhances concrete’s mechanical properties but also helps capture and store up to 30% of atmospheric CO₂ during early curing stages.
Authored by: EF/ DHS
References
- Geetika Mishra, et al., Carbon sequestration in graphene oxide modified cementitious system, Journal of Building Engineering, 2022, 62, 105356;
- Nur Azni Farhana Mazri et al., Graphene and its tailoring as emerging 2D nanomaterials in efficient CO2 absorption: A state-of-the-art interpretative review. Alexandria Engineering Journal, 2023, 77, 479;
- Mohd Hanifa et al., A review on CO2 capture and sequestration in the construction industry: Emerging approaches and commercialised technologies, Journal of CO2 Utilization, 2023, 67, 102292;
- Yating Ye et al., Optimizing the Properties of Hybrids Based on Graphene Oxide forCarbon Dioxide Capture, Ind. Eng. Chem. Res. 2022, 61, 1332;
- Sanglakpam Chiranjiakumari Devi et al., Influence of graphene oxide on sulfate attack and carbonation of concrete containing recycled concrete aggregate, Construction and Building Materials, 2020, 250, 118883