Protecting concrete:
additives and coatings for greater durability in construction
“The compressive strength test is usually the most used parameter as an indicator of concrete quality; however, its value does not determine its durability by itself, that is, in addition to mechanical resistance, permeability and chemical resistance also influence its useful life”
The permeability of concrete is understood as the passage of water and aggressive ions through the capillaries between the aggregates and the cement paste; this is a complex phenomenon and depends above all on the atomic structure of the penetrating ions. One of the most harmful substances for concrete are chloride ions, these can be present from the beginning in the fresh mix, that is, dissolved in the aggregates, additives or in the water, or permeate from the outside, this being the case that exposes the greatest risk of corrosion. Although in general it can be said that the durability of concrete against atmospheric agents depends fundamentally on its permeability to water, while the durability with respect to aggressive salts, both for concrete and for reinforcement, depends on its resistance to the entry of chlorides. by different ways.
“The penetrability of the chlorides is manifested mainly by the diffusion of the ions in the concrete, rather than by the penetration of the entire solution into the samples. That is, the penetration of chlorides does not depend solely on the permeability of the water”
To protect concrete against corrosion, there are two main types of products: on the one hand, there are additives for fresh concrete mixes whose function is to act on the metal surface, canceling the anodic or cathodic reaction or both, and on the other, there are coatings. for the protection of hardened concrete. However, whatever the product used, anticorrosion protection is usually temporary, especially when the structures are subject to movements, loads or temperatures that could affect the performance of the protection or barrier placed.
In the previous article entitled Towards sustainable construction, we discussed the importance of the key nanometric component in the resistance of cement, known as hydrated calcium silicates (C-S-H) or tobermorite gel, and it’s interesting interaction with graphene oxide (GO) nanoparticles, a nanometric structure derived from graphite and of recent interest for the development of more resistant, durable and environmentally friendly structures.
GO is formed by nanometric sheets of carbon atoms linked in a hexagonal pattern and by a series of oxygenated groups anchored to its surface that facilitates its dispersion in water and combining with other materials, for example, with the nanoparticles present in cement (C-S-H).
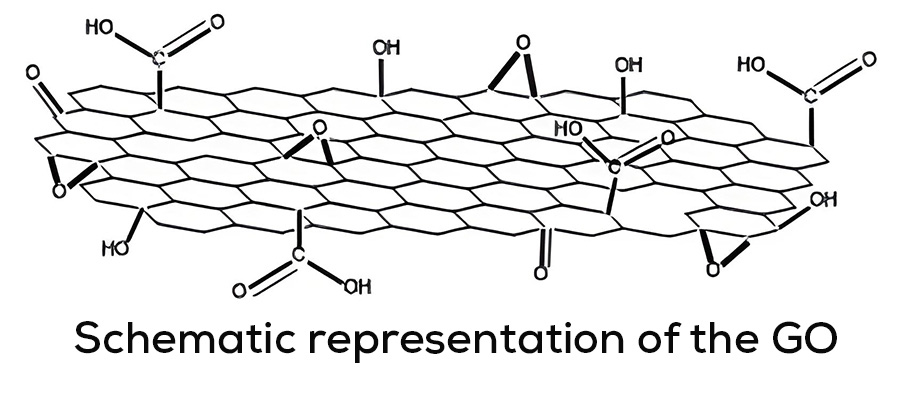
In this regard, international studies show that the shape and surface chemistry of GO allow it to act as a platform to accelerate the hydration of cement and promote the creation of large amounts of C-S-H particles, from the formation of a new GO/ bond. C-S-H. This strong interaction gives rise to a denser network of interlocking cement crystals which, in addition to favoring the mechanical properties of the structures, also acts as a barrier against the infiltration of water through the capillary pores, but with an effect that last longer than currently available additives. This property is extremely important for the durability of concrete and for the prevention of alkali-silica reaction (ASR), an expansion reaction that occurs in the presence of moisture between alkaline cement paste and reactive amorphous silica causing cracks.
Electrical resistivity and corrosion rate Another important test for concrete is electrical resistivity and is defined as the resistance of a material to the passage of electrical charges; its measurement in concrete is a common test to identify the presence of moisture, as well as to predict the initiation period of corrosion in reinforced concrete based on the inverse relationship between electrical resistivity and ion diffusivity. That is, the higher the resistivity, the less movement of electrical charges caused by a lower porosity. The participation of graphene oxide nanoparticles in this property has also been evaluated in different studies that confirm that the GO/C-S-H interaction produces a more compact or less porous concrete that, in addition to reducing water and ion permeability, also limits the movement of electrical charges providing greater anticorrosive protection of metallic concrete structures.
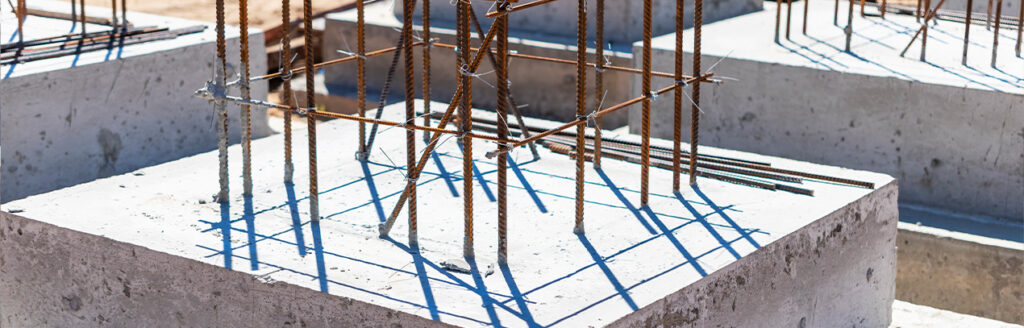
Energeia Fusion (Graphenemex®), the leading Mexican company in Latin America in the research and production of graphene materials, for more than 10 years has been given the task of materializing the benefits of graphene on a scientific basis to turn it into real applications. Thus, after a long journey of research and with results comparable to those reported by various international studies regarding the use of graphene oxide in different products, including concrete, in 2018 it managed to launch Graphenergy Construcción®, the first additive on the market. for concrete with graphene oxide in the world; a multifunctional water-based additive that contributes to improve different properties of cement-based structures with a single application, such as:
- Remodeling of the microstructure of the cement paste with better interfacial bond GO/C-S-H,
- Better compactness of the cement,
- Less movement of electric charges,
- Decrease in the crack extension process,
- Significant reductions in the rate of calcium hydroxide handling,
- Greater mechanical resistance by improving its microstructure,
- Greater durability of the structures due to improvements in impermeability, resistance to chloride penetration and reduction of penetration depth.
It is important to remember that these effects may vary since, in addition to the type of graphene or graphene oxide used, the final properties of cement-based structures also depend on factors such as the water-cement ratio, degree of compaction of the mixture; the characteristics of cement, aggregates, additives, among others, but with proper management and monitoring of graphene additives, the results can be very interesting.
Drafting: EF/DHS
Sources
- Ultrahigh Performance Nanoengineered Graphene- Concrete Composites for Multifunctional Applications. Adv. Funct. Mater. 2018; 28: 1705183;
- The role of graphene/graphene oxide in cement hydration. Nanotechnology Reviews. 2021;10(1): 768;
- Experimental study of the effects of graphene nanoplatelets on microstructure and compressive properties of concrete under chloride ion corrosión. Construction and Building Materials, 2022; 360, 129564;
- Effect Of On Graphene Oxide the Concrete Resistance to Chloride Ion Permeability. IOP Conf. Ser. 2018: Mater. Sci. Eng. 394 032020;
- Effects of graphene oxide on early-age hydration and electrical resistivity of Portland cement paste. Constr Build Mater. 2017; 136, 506;
- Recent progress on graphene oxide for next-generation concrete: Characterizations, applications and challenges. “J. Build. Eng. 2023; 69, 106192;
- Graphene nanoplatelet reinforced concrete for self-sensing structures – A lifecycle assessment perspective. J. Clean. Prod. 2019; 240, 118202;
- Graphene opens pathways to a carbon-neutral cement industry. Science Bulletin. 2021; 67;
- Reinforcing Effects of Graphene Oxide on Portland Cement Paste. J. Mater. Civ. Eng. 2014; A4014010-1;
- A review on the properties, reinforcing effects, and commercialization of nanomaterials for cement-based materials. Nanotechnology Reviews 2020; 9: 303–322, 10;
- Permeabilidad a los cloruros del hormigón armado situado en ambiente marino sumergido. Revista Ingeniería de Construcción. 2007; 22: 1, 15;
- Penetrabilidad del hormigón al agua y a los iones agresivos como factor determinante de su durabilidad. Materiales de Construcción, 1973; 23: 150;
- La resistividad eléctrica como parámetro de control del hormigón y de su durabilidad. Revista ALCONPAT, 2011; 1(2),90;
- Portland cement blended with nanoparticles. Dyna, 2007; 74:152, 277;
- Improvement in concrete resistance against water and chloride ingress by adding graphene nanoplatelet. Cem concr res, 2016; 83: 114