Graphene and nanomedicine:
the perfect combination for improved health
Part III. Dentistry- Implantology
The application of nanotechnology in nanomedicine is based on the fact that most biological molecules, from DNA, amino acids and proteins to constituents such as hydroxyapatite and collagen fibrils, among others, exist and function at the nanometric scale.
Nanometer (nm): millionth of 1 millimeter.
Graphene materials are two-dimensional (2D) sheet-shaped carbon nanoparticles that have gained popularity in the field of biomedical sciences not only for their incredible mechanical, thermal, electrical, optical, and biological properties, but also for their ability to transfer these properties to other materials allowing the possibility of creating new compounds with advanced characteristics. In Odontology, and particularly in relation to implantology, this transfer of properties has opened numerous lines of research with great expectations due to the interesting synergistic effect between infection control and its regenerative capacity.1
Nanoparticle: particle that measures between 1 and 100 nm.
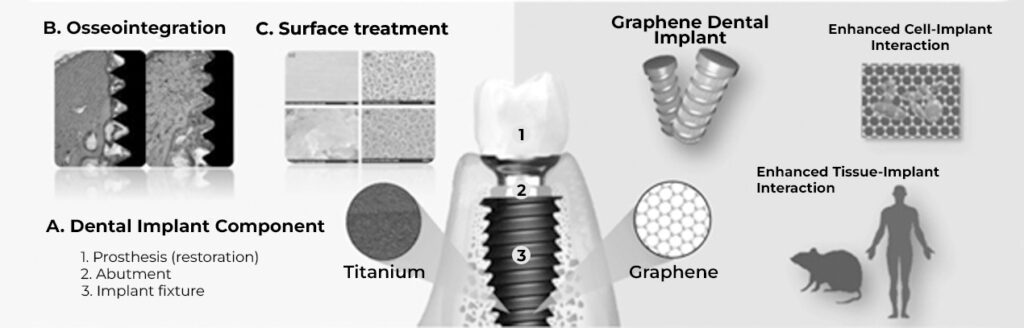
What are the problems that graphene could solve?
Osseointegration
One of the main concerns after the placement of an implant is the failure of its osseointegration. This can occur because instead of bone cells growing at the bone-implant interface, fibrous tissue grows that does not allow implant stabilization. An alternative to favor site conditions where cell interactions will occur is modification of the implant surface by physical or chemical methods to create nanoporosities that increase surface area and favor cell activity. 2
Osseointegration: Firm, stable, and long-lasting connection between an implant and the surrounding bone. Its success depends on biological and systemic factors of the patient, in addition to the characteristics of the implant.
In the case of graphene materials, in addition to their extensive and extremely thin surface area one atom thick, another of their added values is the cloud of electrons that surrounds them, and the presence of some oxygenated groups allows them to interact with proteins serum to form a focal adhesion. In other words, the hydrophobic/hydrophilic nature of these nanomaterials in combination with the roughness of the surface contributes to the interaction with proteins and later with cells, acting as a scaffold to promote the growth, differentiation, and anchorage of bone cells in the implant, paving the way for a stable and predictable osseointegration with a better projection of useful life.3,4
The regenerative impact of graphene materials lies in their great ability to adsorb proteins, creating a layer between cells and the surfaces of the materials to promote cell adhesion and proliferation.1
Infection control
Another cause for implant failure is the appearance of peri-prosthetic or peri-implant infections; to avoid them, it is common to use techniques such as antibiotic impregnation, local drug delivery systems, and the coating of implants with titanium nanotubes, silver nanoparticles, or polypeptide nanofilms for the controlled release of antibiotics.5 However, the worrying increase of antibiotic resistance has made these strategies less and less effective.
Graphene materials, in addition to their biocompatibility, have intrinsic antimicrobial properties with advantages over traditional antibiotics as they have less chance of developing microbial resistance. Odontology has been exploring these effects for several years on bioceramic materials such as alumina and zirconium, metals such as titanium, restorative materials such as glass ionomer, and polymeric materials such as polymethyl methacrylate (PMMA), to name a few. In general, the antimicrobial mechanisms accepted for these nanostructures are: 1) physical damage to the membrane, 2) oxidative stress, 3) inactivation by electron withdrawal, 4) isolation against the passage of nutrients and finally, 5) in the case of coatings, control of hydrophobicity and surface energy can also prevent cell attachment with low affinity and prevent biofilm formation.6,7
Biofilm: Layer of microorganisms that grow and adhere to the surface of a natural structure such as teeth (dental plaque) or artificial such as a medical device (intravascular catheters).
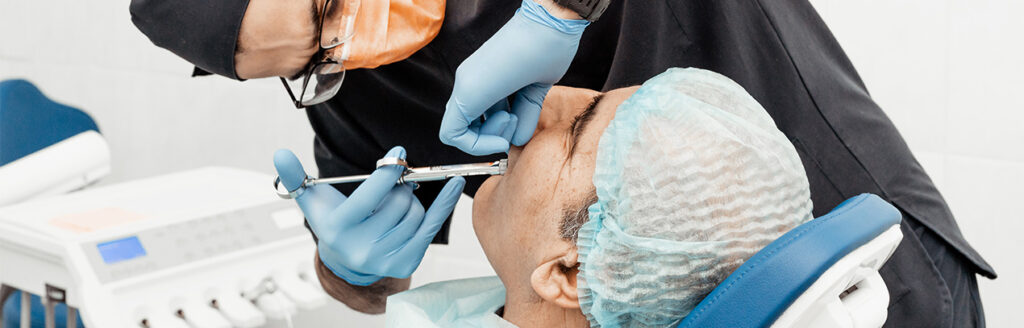
In 2021, a group of scientists from the University of Gwangju, Korea, published a study in which they coated zirconium implants with graphene oxide using the argon plasma method. Their results reported that this modification reduced by 58.5% the presence of Streptococcus mutans, the bacterium with the greatest influence on the formation of dental plaque and dental caries, agreeing with a significant reduction in biofilm thickness of 43.4%. In addition to the antimicrobial effect, they also showed a statistically significant increase of 3.2% and 15.7% in the proliferation and differentiation of bone cells.8 These results are consistent with what was reported by the Jiao Tong University, Shanghai, on a hybrid material of titanium with graphene. synthesized by the spark plasma sintering (SPS) technique. Similarly, the research demonstrated an interesting decrease in the formation of multibacterial biofilms composed of Streptococcus mutans, Fusobacterium nucleatum and Porphyromonas gingivalis, accompanied by an improvement in the activity of human gingival fibroblasts, one of the most important cell groups involved in healing.9 In addition to the synergy between infection control and its regenerative capacity, other studies related to dental implantology are also focusing their attention on the mechanical properties for the design of new implants or restorative materials. 10-12
Energeia-Graphenemex, the pioneering Mexican company in Latin America in the research and development of applications with graphene materials, throughout its 10-year career has overcome numerous scientific and commercial challenges to reach the market with products for different industries. And being aware that to reach the health sector it is essential to carry out exhaustive evaluations, kindly invites all those companies and/or research centers that are interested in continuing to explore the benefits of graphene materials and laying increasingly solid foundations on their safe use for biomedical applications.
Drafting: EF/DHS
References
- ¿Can Graphene Oxide Help to Prevent Peri-Implantitis in the Case of Metallic Implants? Coatings 2022, 12, 1202.
- New design of a cementless glenoid component in unconstrained shoulder arthroplasty: a prospective medium term analysis of 143 cases. Eur J Orthop Surg Traumatol 2013. 23(1):27–34 7.
- European Journal of Orthopaedic Surgery & Traumatology (2018) 28:1257
- Graphene-Based Biomaterials for Bone Regenerative Engineering: A Comprehensive Review of the Field and Considerations Regarding Biocompatibility and Biodegradation. Adv. Healthc. Mater. 2021, 2001414.
- Nanotechnology and bone regeneration: a mini review. 2014 Int Orthop 38(9):1877–1884 /1. European Journal of Orthopaedic Surgery & Traumatology (2018) 28:1257
- Graphene: ¿An Antibacterial Agent or a Promoter of Bacterial Proliferation? iSciencie. 2020. 23, 101787
- Graphene: The game changer in dentistry. IP Annals of Prosthodontics and Restorative Dentistry 2022;8(1):10
- Antibacterial Activity of Graphene Depends on Its Surface Oxygen Content.
- Direct-Deposited Graphene Oxide on Dental Implants for Antimicrobial Activities and OsteogenesisInt. J. Nanomedicine 2021 :16 5745
- Graphene-Reinforced Titanium Enhances Soft Tissue Seal. Front. Bioeng. Biotechnol. 2021. 9:665305.
- Graphene-Doped Polymethyl Methacrylate (PMMA) as a New Restorative Material in Implant-Prosthetics: In Vitro Analysis of Resistance to Mechanical Fatigue. J. Clin. Med. 2023, 12, 1269.
- Mechanical Characterization of Dental Prostheses Manufactured with PMMA–Graphene Composites. Materials 2022, 15, 5391
- Fabrication and properties of in situ reduced graphene oxide-toughened zirconia composite ceramics. J. Am. Ceram. Soc. 2018, 101, 8