Graphene
The Most Versatile Carbon Allotrope with Extraordinary Properties
Carbon is one of Earth’s most abundant elements and vital for living organisms. Known as the “king” of the periodic table, its chemical properties are exceptional due to an electronic structure capable of forming single, double, and triple bonds, allowing it to create up to ten million compounds.
Carbon allotropes are carbon-based materials with different molecular configurations and, consequently, unique properties. For instance, in graphite, a soft, thermally resistant, and electrically conductive material, carbon atoms form three covalent bonds in a hexagonal pattern, arranged in stacked layers loosely bonded together.
Graphite’s common uses include pencils, batteries, and lubricants. Meanwhile, in diamond, an insulating material highly valued in jewelry, carbon atoms are bonded covalently in a tetrahedral structure, giving it extreme hardness used mainly for cutting tools.
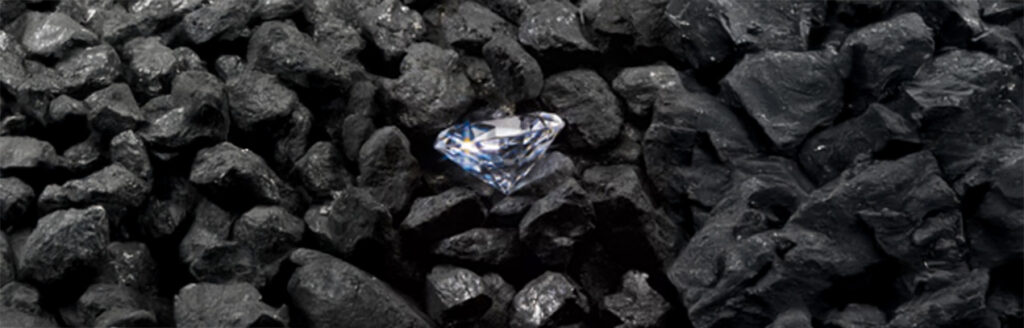
Other lesser-known carbon allotropes are nanometric in size (smaller than 0.1 microns). These include fullerenes, which resemble a soccer ball and can act as semiconductors or superconductors; single- or multi-walled nanotubes, tubular carbon layers known for their strength, elasticity, and conductivity.
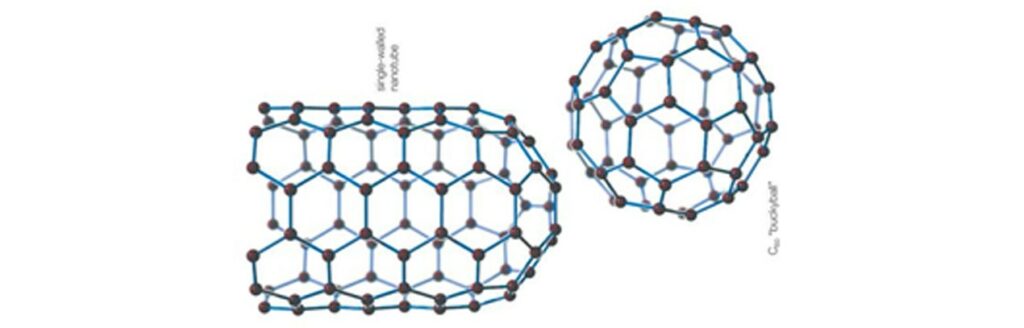
Finally, Graphene is a molecule composed of carbon layers similar to graphite, but in isolated blocks of one to ten layers, offering superior properties in mechanical strength, thermal and electrical conductivity, among others.
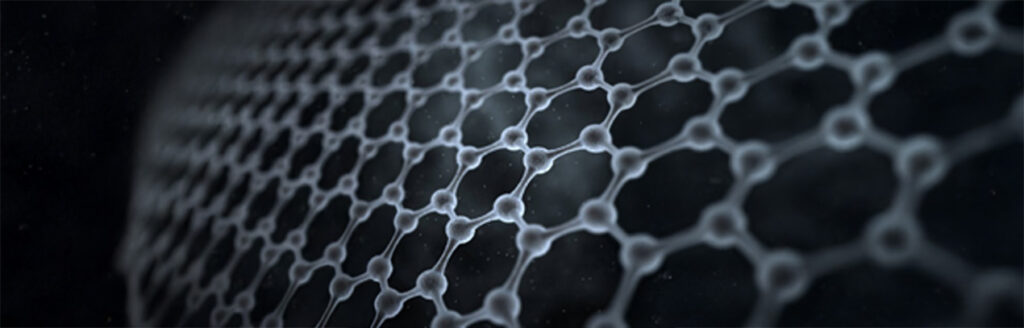
“Other materials should not be classified as carbon allotropes, e.g., activated carbon and carbon black, defined as carbonaceous materials obtained from carbon-containing raw materials.”
Activated Carbon, or charcoal, resembles graphite but has a rough and porous structure with a significant adsorptive capacity, mainly used to remove pollutants in air or water. Unlike graphite or graphene, which have carbon atoms organized in a hexagonal pattern, activated carbon consists of heptagonal and pentagonal rings with disorganized impurities, often produced by carbonizing biomass like wood, coconut shells, bones, or petroleum coke in the absence of air, followed by partial gasification with steam or carbon dioxide to alter its porosity.
“In activated carbon, ‘activation’ refers to the use of physical or chemical means to increase its porosity and surface area.”
Carbon Black, or soot, is an amorphous carbon colloid made of aggregated nanometric spheres with about 1% organic species. It is obtained from the incomplete combustion of hydrocarbons like petroleum under controlled conditions. Although it shares a carbonaceous nature with activated carbon, its properties depend on particle distance rather than porosity. While activated carbon is valued for its adsorptive properties, carbon black is used as a rubber reinforcement, in conductive pigments, or as a UV stabilizer.
What Makes Graphene a Superior Material? Among carbon allotropes and carbonaceous materials, graphene is the most revolutionary nanomaterial and is considered the fundamental unit of all graphite forms, as it can be curved into fullerenes, rolled into nanotubes, or stacked into graphite. Graphene’s superior properties stem from the strong, organized bonds between its atoms, creating a honeycomb structure that explains its mechanical strength, while a free electron from each carbon atom allows its excellent conductivity.
Graphene’s extraordinary multifunctionality extends beyond its mechanical and conductive properties; it is also extremely lightweight, transparent, impermeable, biocompatible, antimicrobial, anticorrosive, radiation-resistant, and can chemically interact with other substances to share its properties. This adaptability promotes its use in various industries, from construction to enhance concrete properties; recycling and plastics to extend material lifespan; anticorrosive and antimicrobial coatings to increase protective efficiency, to electronics, energy, and biomedical fields, offering benefits tailored to each sector’s needs.
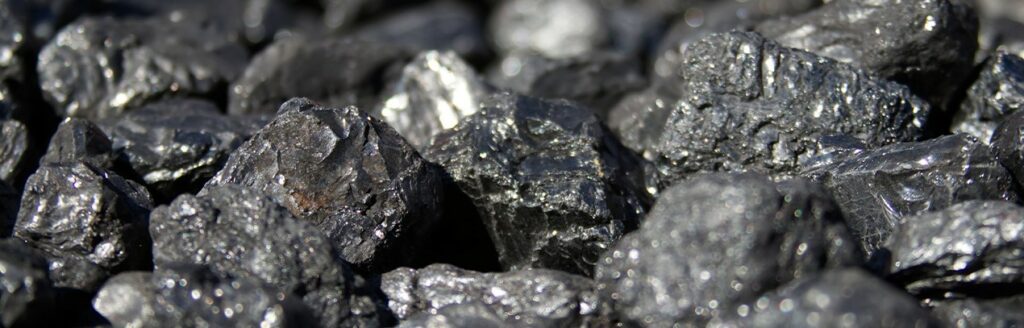
How Is Graphene Produced? There are two main techniques to obtain graphene. The first, known as “bottom-up,” involves Chemical Vapor Deposition (CVD), which extracts carbon atoms from gases like methane. Although well-known, this method is rarely used for industrial production due to low scale and high costs. The second and more common method is “top-down,” involving mechanical, electrochemical, or chemical exfoliation of bulk graphite to isolate carbon or graphene layers. Fewer than 10 layers is considered graphene, while more layers are classified as graphite. Graphene, unlike 3D graphite, has a two-dimensional structure (2D), where thickness is on a nanometric scale. One defining feature is that graphene is just one atom thick.
Energeia-Graphenemex®, a pioneering Mexican company in Latin America focused on graphene material research and production, excels in creating patented methods and processes for scalable graphene production. This ensures availability for developing applications, whether in-house or as a strategic partner with companies interested in innovating and enhancing products with this extraordinary technology.
Written by EF/DHS