Towards a sustainable construction:
how graphene oxide increases the resistance of concrete and favors the reduction of CO2 emissions
The investigation of new technologies for the cement and concrete industry is not only limited to improving its durability, but also to seeking strategies to control its influence on climate change, taking as a background that this industry is the third largest source of emissions of carbon dioxide (CO2) and that the global challenge for 2030 is to reduce these emissions by at least 16%.
Nanotechnology
Nanotechnology for the cement industry is not a new concept, in fact, cement is considered a nanostructured material because 50 to 60% of its composition consists of nanoparticles of approximately 10 nm known as hydrated calcium silicates (C-S-H). or tobermorite gel. This important nanometric component is the foundation for the development of new formulations applying other nanoparticles such as Nano Silica (n. SiO2), Nano Titanium Oxide (n. TiO2), Nano Ferric Oxide (n. Fe2O3), Nano Aluminum Oxide or alumina (n. Al2O3), Clay Nanoparticles and Carbon Nanoparticles, such as carbon nanotubes, graphene nanoparticles or graphene oxide.
“C-S-H fills the empty spaces in the cement, improves the density, cohesion, impermeability and resistance of the cement”
Graphene oxide (GO) is a carbon nanoparticle obtained from the oxidation and exfoliation of graphite. Its well-known mechanical and impermeability properties, combined with a nucleating and densifying effect on the microstructure of cement, captured the attention of scientists and industries when they discovered that the use of low concentrations of this nanomaterial allows the development of more resistant, durable, and friendly structures with the environment.
“GO promotes the formation of C-S-H to improve and accelerate cement hydration through a new chemical bond”
How does GO interact with cement?
Although it is perfectly documented that C-S-H is responsible for 60 to 80% of the strength of cement, recent research has shown that GO further favors these results and that it is not exclusive to improve mechanical strength, but also contributes with other properties such as impermeability, anticorrosiveness and/or thermal insulation. The benefits that GO brings to cement itself or to cement-based materials are attributed to the interaction between the carboxyl groups (COOH) of GO with the C-S-H of the cement to form strong GO/C-S-H chemical bonds. This occurs in the following way, when the cement comes into contact with water, it dissolves and releases large amounts of ions; On the other hand, GO, by presenting a large surface area and good capacity to increase the mobility of Ca2+ ions in the cement paste, GO allows them to be adsorbed on it. In other words, GO acts as a platform to enhance the nucleation or promotion effect to form a large number of C-S-H particles (fig. 1), a phenomenon that ultimately facilitates the hydration process at an early age and which in turn leads to the formation of denser, more resistant and less permeable microstructures.
“Hydration is the process by which cement reacts chemically in the presence of water, develops binding properties and becomes a bonding agent.”
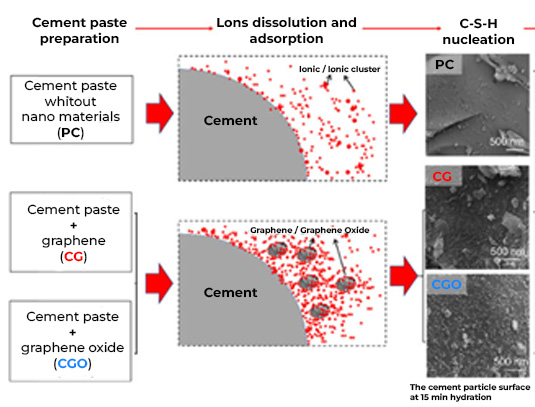
Taken from: Nanotechnology Reviews (2021), vol. 10, no. 1,768
Mechanical resistance vs. lower CO2 emissions
The compressive strength test is the most common measure to control the quality of concrete and, therefore, it is also the most widely used technique to evaluate the effect of GO on these structures. According to tests carried out in the laboratory, the presence of GO in the concrete can exceed 50% of the expected resistance, while field evaluations report improvement fluctuations in the range of 5% to 50%. This variation is due to the fact that, in addition to the type of GO and dosage studied, like any concrete structure, the resistance also depends on factors such as the water-cement ratio, the degree of compaction of the mix; the characteristics of the cement, aggregates and additives; the age of the concrete; the temperature and hygrometry of the curing environment. However, these values are attractive enough to be used not only in the design of more resistant structures, but also with lower cement content to contribute to the reduction of CO2 emissions. In fact, in 2019 a study carried out by researchers from the University of Cambridge revealed that, if the addition of graphene nanoparticles managed to reduce just 5% of the cement in the concrete mix, its effect on global warming would be reduced by one 21%”.
Due to the foregoing and, from the 21st Conference of the Parties to the United Nations Framework Convention on Climate Change (COP 21) held in 2015, which concluded with the adoption of the Decision and the Paris Agreement that, from 2020 promotes low-carbon development to keep the global temperature rise below 2°C, companies from countries such as England, Spain, the United States, Vietnam and Mexico have accelerated their efforts to promote the benefits of graphene nanotechnology in favor of the environment.
Energeia Fusion, the leading Mexican company in Latin America in the production of graphene materials and the development of applications, in 2018 launched Graphenergy Construcción®, the first additive for concrete with graphene oxide in the world; a multifunctional water-based additive that contributes to improving different properties of cement-based structures with a single application. Likewise, in the short term it hopes to have a graphene-reinforced cement available and contribute to achieving environmental commitments.
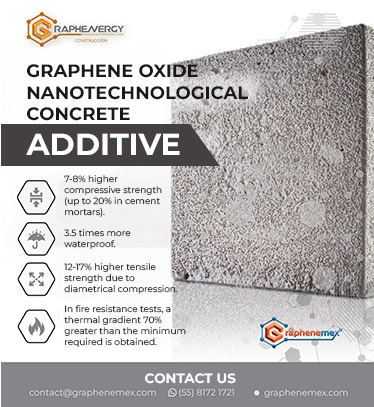
Sources
- Ultrahigh Performance Nanoengineered Graphene- Concrete Composites for Multifunctional Applications. Adv. Funct. Mater. 2018, 28, 1705183;
- The role of graphene/graphene oxide in cement hydration. Nanotechnology Reviews. 2021;10(1): 768;
- Experimental study Construction and Building Materials, 2022, 360, 129564;
- Effect Of On Graphene Oxide the Concrete Resistance to Chloride Ion Permeability. IOP Conf. Ser. 2018: Mater. Sci. Eng. 394 032020;
- Effects of graphene oxide on early-age hydration and electrical resistivity of Portland cement paste. Constr Build Mater. 2017, 136, 506;
- Recent progress on graphene oxide for next-generation concrete: Characterizations, applications and challenges. “J. Build. Eng. 2023, 69, 106192;
- Graphene nanoplatelet reinforced concrete for self-sensing structures – A lifecycle assessment perspective. J. Clean. Prod. 2019, 240, 118202;
- Graphene opens pathways to a carbon-neutral cement industry. 2021, Science Bulletin 67;
- Reinforcing Effects of Graphene Oxide on Portland Cement Paste. J. Mater. Civ. Eng. 2014. A4014010-1;
- A review on the properties, reinforcing effects, and commercialization of nanomaterials for cement-based materials. Nanotechnology Reviews 2020; 9: 303–322, 10;
- Permeabilidad a los cloruros del hormigón armado situado en ambiente marino sumergido. Revista Ingeniería de Construcción. 2007, 22, 1, 15;
- Penetrabilidad del hormigón al agua y a los iones agresivos como factor determinante de su durabilidad. Materiales de Construcción, 1973, 23, 150;
- La resistividad eléctrica como parámetro de control del hormigón y de su durabilidad. Revista ALCONPAT, 2011, 1(2),90;
- Portland cement blended with nanoparticles. Dyna, 2007, 74, 152, 277