The Impact of Graphene on the Plastic Industry:
Innovation and Sustainability
The origins of plastic trace back to 1860 in the United States when Phelan & Collander, amid an ivory shortage—a material widely used for billiard balls, piano keys, jewelry, and decorative structures—announced a call for a material capable of replacing ivory, offering substantial financial compensation for the time. John Wesley Hyatt proposed “celluloid,” a plant-based carbohydrate that, while not fully replacing ivory, became the stepping stone for the development of plastic, with immediate successors like Bakelite and PVC leading to today’s engineering plastics.
The term “plastic” comes from the Greek “plastikos,” meaning “moldable.”
Plastics are synthetic materials obtained through various polymerization processes from petroleum derivatives. Their evolution and refinement have made them essential to numerous industries and activities. However, after years of unchecked use, plastics have become both a solution for many needs and a significant environmental and health issue, as their versatility and demand have also led to increased waste. As a result, the not-so-new philosophy of sustainable circularity, or the circular economy, involves not only awareness of resource use but also economic, infrastructure, and recycling process adaptations.
Recycling involves reprocessing used materials, such as plastics, for reuse. While an excellent tool for preserving natural resources and reducing waste, two key points must be considered. First, recycling doesn’t apply in all cases because not all plastics are recyclable. Second, reprocessing involves stages where materials may lose properties compared to virgin plastics, limiting their use in many industrial applications.
Over the past 20 years, nanotechnology’s intervention in modifying polymers like polyethylene (PE), polypropylene (PP), and polyethylene terephthalate (PET), among others, with carbon nanoparticles like graphene or carbon nanotubes (CNTs), has yielded interesting results regarding improved mechanical, rheological, electrical, and thermal properties. Graphene’s advantage over CNTs, in addition to other intrinsic properties, lies in its sheet-like structure, whose large surface area and greater dispersibility allow it to create more homogeneous phases, improving load transfer and thereby increasing the mechanical strength of modified plastics.
Companies such as Gerdau Graphene (Brazil), Graphenetech S.L. (Spain), Colloids (UK), and Energeia-Graphenemex (Mexico) have positioned various types of graphene-based masterbatches or concentrated plastics in the market over the past five years. Although each company has its own objectives and markets, there are environmental and economic points of convergence that motivated them to improve the plastic industry. Graphene, even in low concentrations (< 2% by weight), can enhance the quality of both virgin and recycled polymers. For example, graphene can increase flexural modulus by 30%, impact resistance by 40%, tensile strength by 17%, and resistance to rupture by 60%. It can also improve resistance to photodegradation. Depending on the specific needs of each development or application, it is possible to restore some of the mechanical properties of recycled plastics and/or extend the material’s lifespan to reduce the circulation of single-use plastics or, alternatively, achieve the same mechanical properties of polymers with reduced thickness.
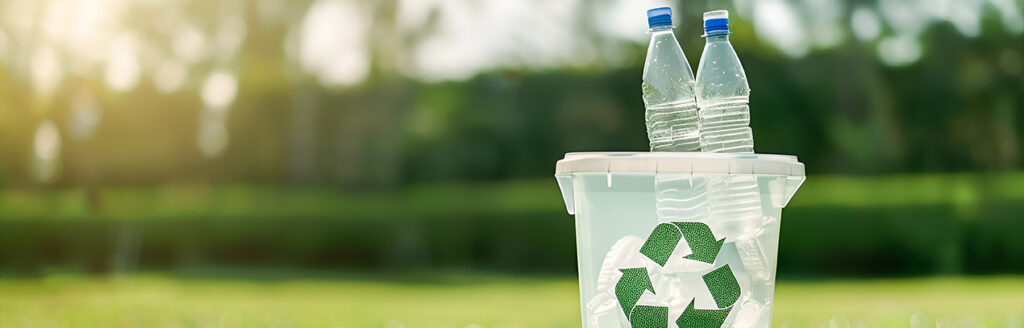
Energeia – Graphenemex®, the leading Mexican company in Latin America in graphene material research and production for industrial applications, launched a wide range of graphene-based masterbatches in 2023 through its Graphenergy Masterbatch line, designed to be used as multifunctional reinforcement additives. Key advantages include:
- Excellent dispersion within the polymer matrix
- Can be incorporated into recycled polymers
- Increase tensile, deformation, and impact resistance
- Improve resistance to ultraviolet rays
- Facilitate processing conditions (thermal stability)
- Act as nucleating agents (modify polymer crystallization temperature).
Drafting: EF/DHS
References:
- Ramazan Asmatulu et al., Synthesis and Analysis of Injection-Molded Nanocomposites of Recycled High-Density Polyethylene Incorporated With Graphene Nanoflakes, POLYMER COMPOSITES—2015;
- Feras Korkees et al., Functionalised graphene effect on the mechanical and thermal properties of recycled PA6/PA6,6 blends. 2021 Journal of Composite Materials 55(16);
- Devinda Wijerathne et. al., Mechanical and graphe properties of graphene nanoplatelets-reinforced recycled polycarbonate composites. International Journal of Lightweight Materials and Manufacture 6 (2023) 117e128;
- Abdou Khadri Diallo et al., A multifunctional additive for sustainability, Sustainable Materials and Technologies, 33, 2022, e000487.